Quantum computers can work millions of times faster than regular computers, but they need a separate network for quantum communications for long-distance communication. Researchers at NASA’s Jet Propulsion Laboratory and Caltech have created a device that can accurately count a large number of single photons, which are quantum particles of light, so they can set up this kind of network. The Performance-Enhanced Array for Counting Optical Quanta (PEACOQ) detector is like measuring individual drops of water being sprayed by a firehose. It can tell within 100 trillionths of a second when each photon hits at a rate of 1.5 billion photons per second. No other detector can match this rate of finding things.
Ioana Craiciu, a postdoctoral scholar at JPL and a PEACOQ project team member, is the study’s lead author. He talks about these results: “Transmitting quantum information over long distances has, so far, been very limited,” Moreover, he said. “A new detector technology like the PEACOQ that can measure single photons with a fraction of a nanosecond precision enables sending quantum information at higher rates, farther.”
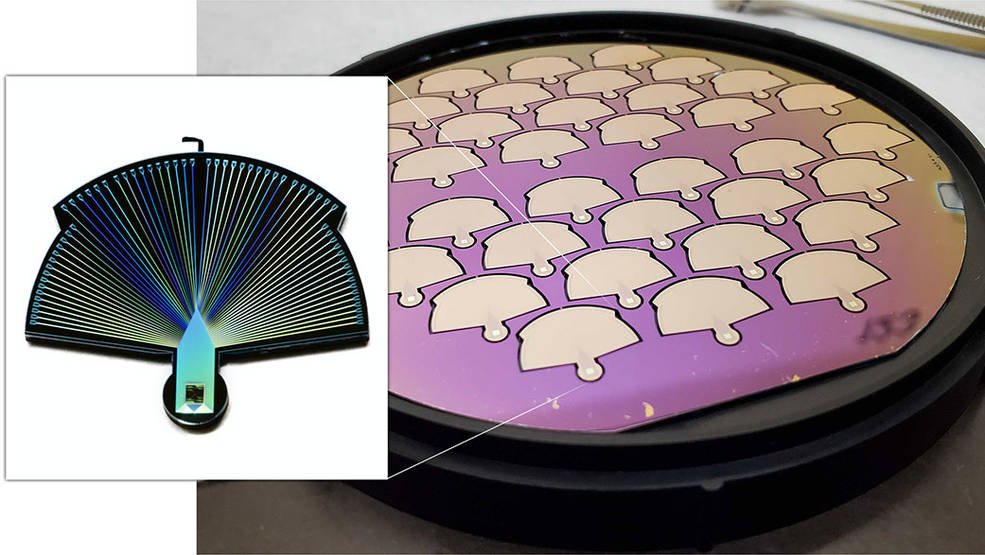
A dedicated Network is Required:

To resolve these problems, quantum computers must talk to each other through a free-space optical quantum network. This network uses satellites in orbit to transmit data. These satellites create entangled photon pairs, which are then sent to quantum computer terminals on Earth, located hundreds or thousands of miles apart.
Superconducting Plumage:
The detector is so tiny that it only takes up 13 microns of space. It comprises 32 superconducting nanowires made of niobium nitride placed on a silicon chip and linked by wires that spread like a bird’s feathers. Each nanowire is 10,000 times thinner than a single human hair.
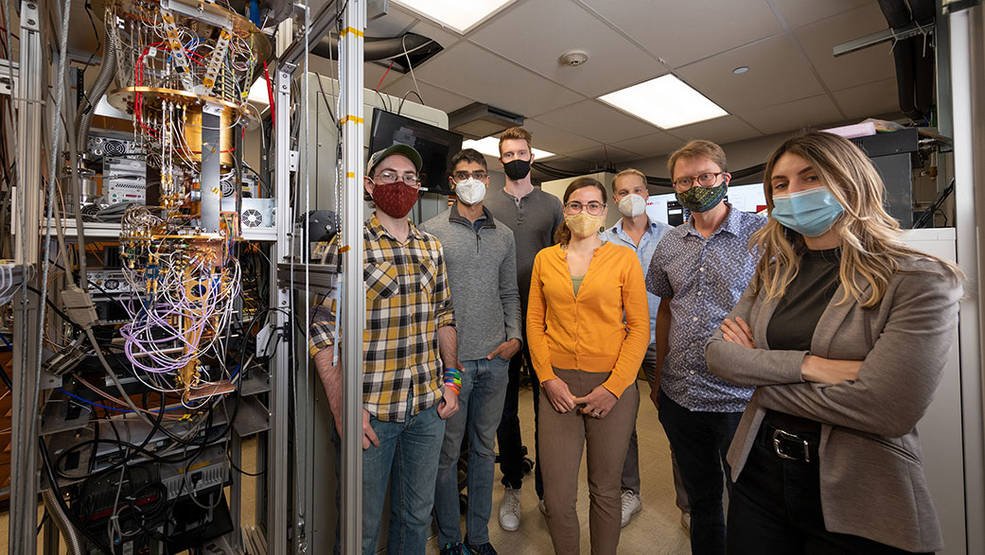
NASA/JPL-Caltech
The Space Operations Mission Directorate’s Space Communications and Navigation (SCaN) program paid for developing the PEACOQ detector. The Microdevices Laboratory at JPL made it. To maintain the nanowires in a superconducting state, the detector must operate at a cryogenic temperature of only one degree above absolute zero, which is -458 degrees Fahrenheit (-272 degrees Celsius). This allows nanowires to convert photons into quantum data-carrying electrical pulses.
The PEACOQ detector has to be able to find a single photon, but it also has to handle multiple photons hitting it simultaneously. When a photon hits a nanowire in a detector, the wire can’t pick up another photon quickly. This is called “dead time.” To minimize dead time, each nanowire in the PEACOQ detector is designed for high efficiency. The detector incorporates 32 nanowires, allowing it to continue capturing photons with other wires while one is in slow time.
“In the near term, PEACOQ will be used in lab experiments to demonstrate quantum communications at higher rates or over greater distances,” Further says. “In the long term, it could provide an answer to the question of how we transmit quantum data around the world.”

NASA/JPL-Caltech
Deep Space Test:
PEACOQ is part of NASA’s broader initiative to establish free-space optical communications between space and the Earth. This device is based on the detector created for NASA’s Deep Space Optical Communications (DSOC) technology demonstration. Scheduled for a 2023 launch with the Psyche mission, DSOC will demonstrate high-bandwidth optical communications between Earth and deep space. Although it won’t transmit quantum information, DSOC’s ground terminal at Caltech’s Palomar Observatory needs extreme sensitivity to detect single photons from its transceiver, sent through deep space by lasers.
JPL’s Matt Shaw advises the superconducting detector team: “It’s all kind of the same technology with a new category of the detector,” Moreover, he said. “Whether we are encoding that photon with quantum information or detecting single photons from a laser source in deep space, we are still counting single photons.”