Introduction to Protoplanetary Disk Studies and Webb’s Role
The study of planet formation is a cornerstone of contemporary astrophysics, offering insights into the origins of our solar system and the potential for life-bearing planets elsewhere. NASA’s James Webb Space Telescope (JWST) represents a paradigm shift in this domain, providing unprecedented clarity on the processes shaping planetary genesis.
Why is planet formation important?
Planet formation is a fundamental process in astrophysics and planetary science, offering critical insights into a variety of scientific and philosophical inquiries. The significance of planet formation extends across several dimensions:
- Understanding Solar System Origins: The study of planet formation provides a narrative for how our own solar system came into being, explaining the current arrangement of planets, asteroids, and comets, as well as the existence of various surface features and atmospheric compositions.
- Exoplanetary Science and the Search for Life: Discovering how planets form enhances the search for exoplanets and the assessment of their potential to harbor life. By understanding the conditions conducive to planet formation, scientists can better predict where habitable planets might be found.
- Astrochemical Processes: Planet formation is deeply tied to the chemical evolution of the universe. The process by which simple dust and gases transform into complex planetary bodies involves a myriad of chemical reactions and physical conditions that also contribute to the synthesis of organic compounds, some of which are the building blocks for life as we know it.
- Cosmic Dust and Elemental Abundance: Planetary formation is one of the main processes that dictate the distribution of elements and compounds in a galaxy. The remnants of planet formation, such as asteroids and comets, can offer a window into the primordial conditions of the early solar system, providing a record of the distribution of elements and isotopes during the solar system’s infancy.
- Planetary Dynamics and Stability: Understanding how planets form sheds light on the dynamics of planetary systems, including orbital mechanics, stability, and the long-term evolution of planetary orbits, which influence a planet’s climate and geological history.
- Interdisciplinary Connections: The complexities of planet formation intertwine with other fields, such as geology, chemistry, and biology. For instance, the geological diversity on Earth and other planetary bodies is a direct consequence of the processes that occurred during their formation.
- Philosophical and Cultural Implications: On a broader cultural and philosophical level, understanding planet formation stirs fundamental questions about humanity’s place in the universe, the uniqueness of our planet, and the potential for other life-forming environments.
Thus, studying planet formation is not only about comprehending the past but also about projecting possible futures in space exploration, planetary habitation, and the search for extraterrestrial intelligence. It is a profound and necessary pursuit for advancing human knowledge and for satisfying the innate curiosity about our cosmic origins and destiny.

NASA, ESA, CSA, Joseph Olmsted (STScI)
Theoretical Background of Planet Formation
Planet formation theories posit that protoplanetary disks—rotating accumulations of gas and dust around young stars—are the birthplaces of planets. Icy pebbles within these disks are hypothesized to migrate inward, seeding the formation of rocky planets and delivering essential volatiles such as water.
What are the 5 steps of planet formation?
The five main stages of planet formation, as understood from current astrophysical models, are as follows:
- Nebular Contraction and Disk Formation: This initial stage involves the collapse of a molecular cloud, which is a dense region of gas and dust in a galaxy. The collapse may be triggered by a variety of events, including the shockwave from a nearby supernova. As the cloud collapses under its own gravity, it begins to spin faster and flattens into a rotating protoplanetary disk around a new star, with the future planets forming from this disk.
- Dust Coagulation and Planetesimal Formation: Within the protoplanetary disk, dust particles collide and stick together through electrostatic forces and other bonding mechanisms, growing from microscopic sizes to pebbles and then to larger bodies known as planetesimals, which are typically kilometers in size. This process is facilitated by the relatively calm conditions within the mid-plane of the disk.
- Accretion of Protoplanets: Planetesimals accumulate more mass through further collisions and accretion, leading to the formation of protoplanets. These bodies are large enough to begin to exert a significant gravitational force, pulling in more material and potentially clearing paths in the disk. During this phase, differentiation occurs, where denser materials sink to the center and lighter materials form an outer crust.
- Gas Capture and Giant Planet Formation: For the formation of gas giants like Jupiter and Saturn, a protoplanet must grow large enough for its gravity to capture the surrounding hydrogen and helium before the star’s radiation blows these light gases away. This process of capturing and accumulating a thick atmosphere of gas can only happen beyond the snowline, where ice can also contribute to rapid growth.
- Late Heavy Bombardment and Planetary Migration: The final stage of planet formation is characterized by a period known as the Late Heavy Bombardment, during which planets and planetesimals are subjected to intense collisions, further shaping their mass and size. Additionally, interactions with residual disk material and other bodies can cause newly formed planets to migrate from their original orbits. This period refines the architecture of the planetary system into a more stable configuration.
Observational Breakthroughs with the James Webb Space Telescope
The JWST has, for the first time, provided empirical evidence of water vapor within protoplanetary disks, corroborating the hypothesis that inward-drifting ice-coated solids are instrumental in planet formation. These observations confirm the existence of cold water vapor released as the icy pebbles surpass the snowline, transitioning from a frozen to a gaseous state.
Methodology of Webb’s Water Vapor Observation in Protoplanetary Disks
Using Webb’s MIRI, researchers analyzed the mid-infrared spectrum of four protoplanetary disks to discern the presence and distribution of water vapor. This selection of disks offered a cross-sectional analysis of the varied environments where planet formation is hypothesized to occur.
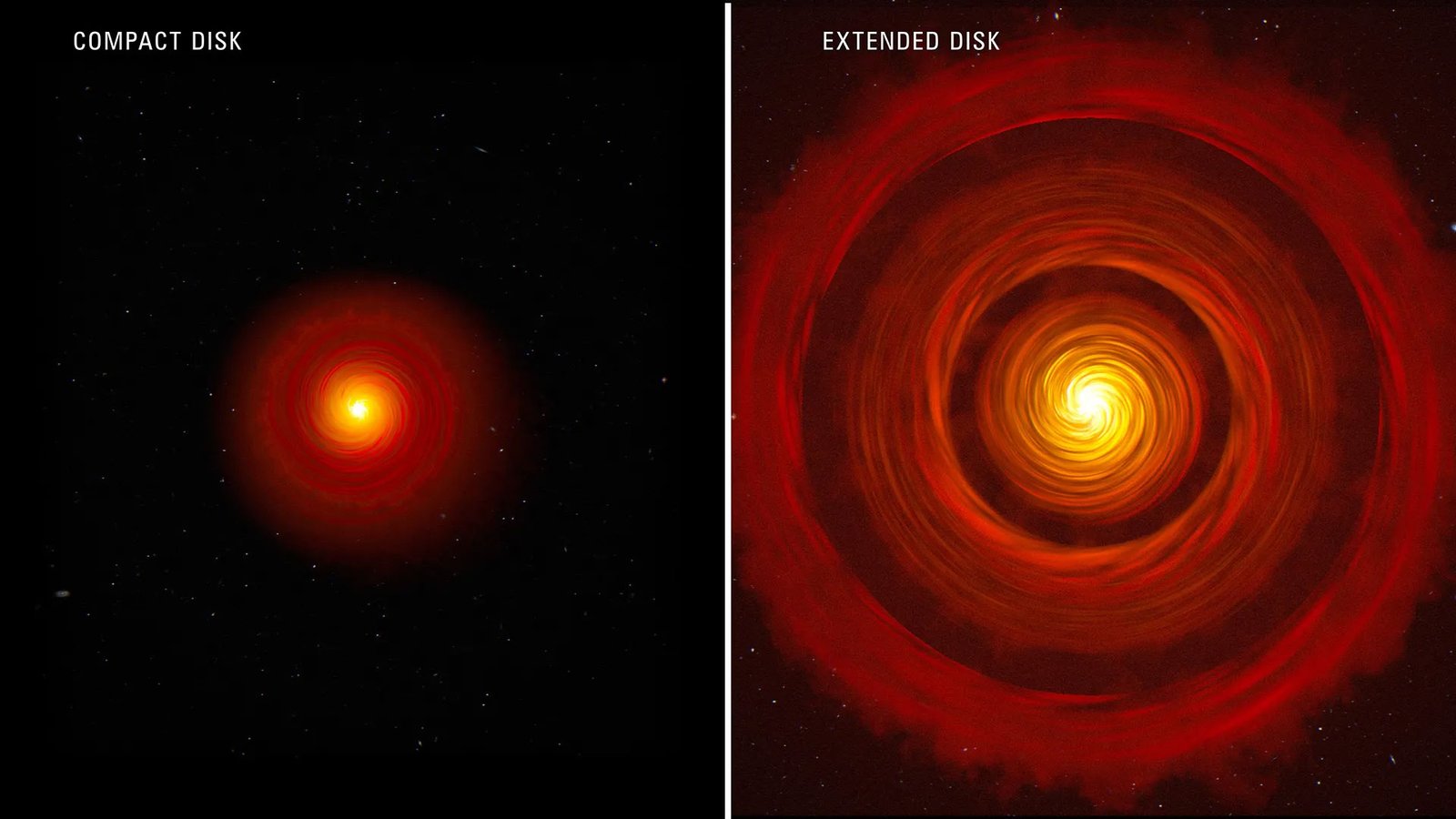
NASA, ESA, CSA, Joseph Olmsted (STScI)
Interpretation of Webb’s Observations and their Implications for Planet Formation
Webb’s observations exhibited a discernible variance in water vapor content between compact and extended disks. The compact disks displayed an abundance of cool water vapor, indicating efficient pebble drift into their inner regions. In contrast, extended disks retained pebbles in outer rings, suggesting a less dynamic inward migration.

NASA, ESA, CSA, Leah Hustak (STScI)
The Phenomenon of Pebble Drift and Snowline Dynamics
Pebble drift is a process governed by the interaction between solid particles and the gaseous component of the disk. The snowline demarcates a thermal boundary within the disk, beyond which ice can form on pebbles. As pebbles migrate through this boundary, the transition of ice into vapor marks a critical phase in planet formation.
What factors affect planet formation?
Planet formation is influenced by a confluence of astrophysical factors, each contributing to the diversity and characteristics of planetary systems. These factors include:
- Stellar Mass and Metallicity: The mass and chemical composition of the progenitor star significantly influence the process of planet formation. High metallicity—referring to the abundance of elements heavier than hydrogen and helium—tends to correlate with a higher probability of forming terrestrial planets due to the greater availability of solid material.
- Properties of Protoplanetary Disk: The mass, extension, temperature, and density profile of the protoplanetary disk govern the material distribution and the time available for planet formation. The disk’s lifetime before dissipation due to starlight and stellar winds also determines the potential for planet growth.
- Dynamics within the Disk: Turbulence, spiral arms, and pressure variations within the disk can create regions where material accumulates or dissipates, impacting the formation of planetesimals and the accretion of protoplanets.
- Presence and Position of Snow Line: The location in the disk where temperatures drop sufficiently for volatile compounds like water to freeze (known as the snow line) is crucial. Beyond this line, ice can enhance the growth of planetesimals and facilitate the rapid accretion of gas giants.
- Angular Momentum and Orbital Resonance: The distribution of angular momentum within the disk affects the speed and direction of material accretion, while orbital resonances between forming planets can alter their orbits, promoting or inhibiting further growth.
- Impact of Giant Planets: The formation of gas giants like Jupiter can have a profound impact on the formation of other planets, as their large masses can stir up the disk, shepherd material, and even disrupt the growth of other potential planetary bodies.
- External Forces: Gravitational interactions with nearby stars, passing stars, or the galactic environment can disturb the disk, truncate its edges, or strip away material, affecting the eventual outcome of the planet formation process.
- Initial Conditions of the Molecular Cloud: The initial angular momentum, mass distribution, and temperature of the molecular cloud that collapses to form the star and disk set the stage for the entire planet formation process.
- Timing and Sequence of Formation Events: The sequence in which various stages occur can result in different planetary systems. Early formation of giant planets, for instance, can prevent the accumulation of sufficient material to form other large planets.
- Migration: Planetary migration, due to interactions with the protoplanetary disk or other forming planets, can redistribute potential planet-building material, altering the kinds of planets that form and where they end up in the planetary system.
These factors interact in complex ways, leading to the formation of a wide variety of planetary systems observed by astronomers. Research in this field is dynamic, with ongoing discoveries continually refining our understanding of how planets are made.
The Role of Pressure Bumps and Pressure Traps in Disk Structure
Pressure bumps within disks act as barriers to pebble drift, creating regions where solids can accumulate. These features may arise from the gravitational influence of forming planets or variations in disk density and temperature.
Comparative Analysis of Compact and Extended Disks
The comparison between compact and extended disks is crucial to understanding the efficiency of pebble drift and water delivery mechanisms. The observed excess of cooler water in compact disks is indicative of a more active inward delivery of materials necessary for the formation of terrestrial planets.
Impact on Understanding of Solar System Evolution
The data from Webb’s telescope offer new perspectives on the evolutionary history of our own solar system, where Jupiter may have played a similar role in shaping the inner planetary system’s composition by acting as a barrier to pebble accretion.
Future Prospects and Directions for Research
These findings pave the way for further exploration of protoplanetary disks and the processes by which planets form and evolve. Future studies may focus on the detailed chemical composition of these disks, the role of other volatiles in planetary development, and the direct observation of planet formation in action.
Conclusion
The confirmation of inward pebble drift and water vapor delivery by the JWST marks a significant advancement in the understanding of planetary formation. This discovery aligns with predictions of planet formation theories and reinforces the dynamic and interconnected nature of these celestial phenomena. As a result, the JWST not only enhances our comprehension of planetary genesis but also amplifies the potential for discovering new, life-supporting worlds.